Kevin Duff
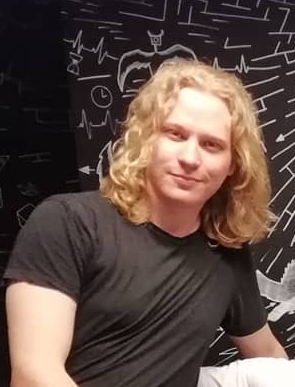
Kevin Duff
Member of Robinson College
PhD student in Prof Payne's group
Office: 515 Mott Bld
Phone: +44(0)1223 3 37460
Email: kkbd2 @ cam.ac.uk
TCM Group, Cavendish Laboratory
19 JJ Thomson Avenue,
Cambridge, CB3 0HE UK.
Research
The physical and chemical properties of transition metal nanoparticles are of great interest for several industrial applications, for example in catalytic reactions. These properties are often very different to those of the bulk crystal and in order to investigate them an accurate description of the electronic structure is required. This is made possible with density functional theory (DFT), but traditional DFT methods suffer from unfavourable cubic scaling. This limits the size of system that can be feasibly investigated to the few thousand atoms, while many useful investigations contain systems on the many-thousand atom scale (e.g. a metallic nanoparticle sitting on a support surface). Linear-scaling approaches such as the one used by ONETEP (www.onetep.org) allow for the study of systems containing tens or even hundreds of thousands of atoms but only apply to systems containing a band gap.
The goal of my PhD is to perform investigations into catalytic reactions that are currently feasible with traditional DFT methods while also preparing ONETEP to be able to handle larger-scale investigations. This has involved extending ONETEP's ability to simulate magnetic metals as well as implementing robust and scaleable transition state tools. As a result, ONETEP is now able to perform investigations of chemical processes on catalyst particles not feasible with traditional DFT codes.
In Plain English
Metallic nanoparticles are important to industry as they act as catalysts to many reactions. It is difficult to probe the exact behaviour of these particles in reactors experimentally, so we turn to highly accurate computer simulation based on quantum mechanics. Density functional theory is an approach that makes the simulation of increasingly complex materials feasible, and using it we are now able to do things like predict new materials and probe the function and mechanisms of biomolecules that are composed of many thousands of atoms entirely computationally. However, the properties of quantum mechanics that we can exploit to simulate very large systems such as biomolecules don't apply to metallic systems, and our ability to simulate large metal structures such as nanoparticles is currently limited. My work involves performing calculations on metals that are currently feasible while pushing that boundary and exploring new methods to access larger length scales.